I just stumbled across an amazing – albeit a little obscure – fact: if you ever drop a gummy bear into potassium chlorate, be prepared for a big bang!
The experiment is simple. Put a small amount of potassium chlorate into a glass test tube, and gently heat it. Now drop in a single gummy bear – and stand behind a protective shield. The gummy bear will burst into a flame-shooting reaction for about a minute.
Don’t believe me? You can see an example of this at:
http://blog.wired.com/wiredscience/2008/01/video-gummy-bea.html
(I really recommend watching this video. It’s amazing how many flames a single gummy bear will produce!)
So I have 2 questions about this flaming gummy phenomenon. (1) Why does it happen? And (2) Why should I care?
First, why does it happen? Potassium chlorate is a powerful oxidizer – a substance that burns when you give it a combustible fuel, since it easily gives up oxygen to fuel the reaction. In this case, the combustible fuel is the sugar in the gummy bear. Sugar is a molecule that stores a lot of energy. When that molecule is oxidized rapidly, all of the energy gets released in one quick step. So the amount of energy released by the potassium chlorate is the same as what you would get by metabolizing the gummy bear in your body – the energy is just released more quickly.
So mix the 2 together, and the potassium chlorate releases oxygen molecules, which oxidize the sugar, producing energy in the form of lots of big flames. It’s that simple!
So why should I care? I think it’s a great example of how to illustrate simple chemical concepts in a really fun manner. The concepts here – the amount of energy stored in sugar, and the process of oxidation. If you were to just describe how sugar is a great holder of energy, and oxidizing agents can burn spontaneously, it sounds kind of boring. But show someone a picture of a gummy bear bursting into flames, and all of a sudden science becomes much more fun!
Thursday, January 31, 2008
Thursday, January 24, 2008
Serious – and not so serious – research
The Nobel Prize in Medicine. A serious award about serious science. Nobel laureates have made a significant, lasting, and important contribution to our understanding of the scientific world, work that has dramatically changed what we know about how our world works and how we conduct research. For example:
In 2006, Andrew Fire and Craig Mello were awarded the Nobel for the discovery of RNA interference - gene silencing by double-stranded RNA. This is an experimental technique used by thousands of scientists today.
In 2002, Sydney Brenner, H. Robert Horvitz and John E. Sulston won the prize for the genetic regulation of organ development and programmed cell death. We now understand that cells have dedicated mechanisms not only to keep themselves alive, but also to kill themselves when necessary.
In 1999, Gunter Blobel received his Nobel prize for learning that proteins have intrinsic properties that dictate their movement and final place within a cell. This property forms the basis of a huge field of current biological research – protein trafficking.
And in 1993, Richard Roberts and Phillip Sharp were given the Nobel for discovering split genes. It had previously been thought that genes were always one long, continuous unit. Drs. Roberts and Sharp clarified that genes are often broken into multiple sections, which get cut and pasted back together in a process called splicing. This presented a dramatic shift in the understanding of how genes are regulated.
Yes, the Nobel prizes are inspiring and dramatic. They are the serious side of research. But there is also a less-than-serious award about less-than-serious science, which I personally find highly entertaining. An award ceremony held every year celebrates the not-so-inspiring research, the research that doesn’t really change our world, but (quite often) the research that makes us laugh – and hopefully remember why we love science. They’re called the Ig Nobels.
The Ig Nobels are awarded by an organization called Improbable Research. Their stated goal: “The Ig Nobel Prizes honor achievements that first make people laugh, and then make them think. The prizes are intended to celebrate the unusual, honor the imaginative -- and spur people's interest in science, medicine, and technology.” To give you an idea of the kinds of research that are awarded Ig Nobels, here are a few examples of the winners for 2007. (Remember, this research is all actual research, published in real, peer-reviewed scientific journals.)
In Medicine: Brian Witcombe and Dan Meyer – Sword swallowing and its side effects. Based on: “Sword Swallowing and its Side Effects” published in the British Medical Journal
In Physics: L. Mahadevan and Enrique Cerda Villablanca - How sheets become wrinkled. Based on: “Wrinkling of an Elastic Sheet Under Tension” published in Nature
In Chemistry: Mayu Yamamoto – Extracting vanilla from cow dung. Based on: “Novel Production Method for Plant Polyphenol from Livestock Excrement Using Subcritical Water Reaction”, internally published in the International Medical Center of Japan
In Linguistics: Juan Manuel Toro and Nuria Sebastian-Galles – Rats sometimes cannot tell the difference between a person speaking Japanese backwards and a person speaking Dutch backwards. Based on: "Effects of Backward Speech and Speaker Variability in Language Discrimination by Rats," published in the Journal of Experimental Psychology
In fact, if you look back at all the Ig Nobels awarded over the last 10 years, you’ll find a variety of topics that will probably make you sit up and say – “someone studied that?!?” This includes the attraction of mosquitoes to limburger cheese, how herrings communicate with flatulence, calculating the surface area of an elephant, the levitation of frogs with magnets, helping make clams happier by giving them Prozac, and whether humans can swim faster in water or syrup. Wow. Now that’s some funny science!
My personal favorite, though, has to be the 2004 award for psychology. It was awarded to Daniel Simons and Christopher Chabris for demonstrating that when people pay close attention to something, it's all too easy to overlook anything else.
Dr. Simons and Dr. Chabris made a video of a group of 6 people tossing a basketball amongst themselves. In the middle of the video, a person in a gorilla suit walks into the middle of the circle of people. The video was shown to test subjects, who were told to count the number of times the basketball changed hands. After they watched the video, they were asked about what else they saw besides the basketball. And here’s what’s really funny. An astonishingly large percent of the test subjects did not notice the person in the gorilla suit. They were so focused on the task at hand – counting the passes of the ball – that they completely failed to see a six-foot tall person walking around in a gorilla suit. Now that’s what I call focus!
Now, the Ig Nobels may seem silly and inane. But I think that they serve a very important function. Science is serious. It’s significant. It can be intense. But science is also supposed to be fun. It’s supposed to intrigue and excite people. It’s supposed to make you think about things you never knew before. It’s supposed to stimulate your imagination. And what better way to make science fun than to show people a video with someone walking around in a gorilla suit!
By the way, if you want to watch the basketball and gorilla video yourself, go to: http://viscog.beckman.uiuc.edu/media/ig.html
Click on the link “View the “basketball” video." I hope it makes you laugh!
In 2006, Andrew Fire and Craig Mello were awarded the Nobel for the discovery of RNA interference - gene silencing by double-stranded RNA. This is an experimental technique used by thousands of scientists today.
In 2002, Sydney Brenner, H. Robert Horvitz and John E. Sulston won the prize for the genetic regulation of organ development and programmed cell death. We now understand that cells have dedicated mechanisms not only to keep themselves alive, but also to kill themselves when necessary.
In 1999, Gunter Blobel received his Nobel prize for learning that proteins have intrinsic properties that dictate their movement and final place within a cell. This property forms the basis of a huge field of current biological research – protein trafficking.
And in 1993, Richard Roberts and Phillip Sharp were given the Nobel for discovering split genes. It had previously been thought that genes were always one long, continuous unit. Drs. Roberts and Sharp clarified that genes are often broken into multiple sections, which get cut and pasted back together in a process called splicing. This presented a dramatic shift in the understanding of how genes are regulated.
Yes, the Nobel prizes are inspiring and dramatic. They are the serious side of research. But there is also a less-than-serious award about less-than-serious science, which I personally find highly entertaining. An award ceremony held every year celebrates the not-so-inspiring research, the research that doesn’t really change our world, but (quite often) the research that makes us laugh – and hopefully remember why we love science. They’re called the Ig Nobels.
The Ig Nobels are awarded by an organization called Improbable Research. Their stated goal: “The Ig Nobel Prizes honor achievements that first make people laugh, and then make them think. The prizes are intended to celebrate the unusual, honor the imaginative -- and spur people's interest in science, medicine, and technology.” To give you an idea of the kinds of research that are awarded Ig Nobels, here are a few examples of the winners for 2007. (Remember, this research is all actual research, published in real, peer-reviewed scientific journals.)
In Medicine: Brian Witcombe and Dan Meyer – Sword swallowing and its side effects. Based on: “Sword Swallowing and its Side Effects” published in the British Medical Journal
In Physics: L. Mahadevan and Enrique Cerda Villablanca - How sheets become wrinkled. Based on: “Wrinkling of an Elastic Sheet Under Tension” published in Nature
In Chemistry: Mayu Yamamoto – Extracting vanilla from cow dung. Based on: “Novel Production Method for Plant Polyphenol from Livestock Excrement Using Subcritical Water Reaction”, internally published in the International Medical Center of Japan
In Linguistics: Juan Manuel Toro and Nuria Sebastian-Galles – Rats sometimes cannot tell the difference between a person speaking Japanese backwards and a person speaking Dutch backwards. Based on: "Effects of Backward Speech and Speaker Variability in Language Discrimination by Rats," published in the Journal of Experimental Psychology
In fact, if you look back at all the Ig Nobels awarded over the last 10 years, you’ll find a variety of topics that will probably make you sit up and say – “someone studied that?!?” This includes the attraction of mosquitoes to limburger cheese, how herrings communicate with flatulence, calculating the surface area of an elephant, the levitation of frogs with magnets, helping make clams happier by giving them Prozac, and whether humans can swim faster in water or syrup. Wow. Now that’s some funny science!
My personal favorite, though, has to be the 2004 award for psychology. It was awarded to Daniel Simons and Christopher Chabris for demonstrating that when people pay close attention to something, it's all too easy to overlook anything else.
Dr. Simons and Dr. Chabris made a video of a group of 6 people tossing a basketball amongst themselves. In the middle of the video, a person in a gorilla suit walks into the middle of the circle of people. The video was shown to test subjects, who were told to count the number of times the basketball changed hands. After they watched the video, they were asked about what else they saw besides the basketball. And here’s what’s really funny. An astonishingly large percent of the test subjects did not notice the person in the gorilla suit. They were so focused on the task at hand – counting the passes of the ball – that they completely failed to see a six-foot tall person walking around in a gorilla suit. Now that’s what I call focus!
Now, the Ig Nobels may seem silly and inane. But I think that they serve a very important function. Science is serious. It’s significant. It can be intense. But science is also supposed to be fun. It’s supposed to intrigue and excite people. It’s supposed to make you think about things you never knew before. It’s supposed to stimulate your imagination. And what better way to make science fun than to show people a video with someone walking around in a gorilla suit!
By the way, if you want to watch the basketball and gorilla video yourself, go to: http://viscog.beckman.uiuc.edu/media/ig.html
Click on the link “View the “basketball” video." I hope it makes you laugh!
Tuesday, January 22, 2008
Laundry science
Have you ever wondered how dryer sheets work?
I have. I tend to collect static electricity - my hair, my clothes, even my cats. So I absolutely have to have a dryer sheet in the dryer whenever I’m doing a load of laundry. If I don’t, I’m doomed to be a crackling, zapping, static-y mess. So in goes a dryer sheet, and out comes nice, static-free laundry. It’s like magic!
Well, actually, it isn't magic, it's science. Here’s how they work.
First, let’s talk about static electricity. Static electricity occurs when an electrical charge builds up on a surface. Electrical charge is made of electrons. (“Electrons” and “electricity” sound so similar because they are from the same root.) What are electrons? They are one of three major particles that make up atoms, along with protons and neutrons. The names of these things refer to the electrical charge that they carry: neutrons are neutral – not charged; protons have a positive charge; electrons have a negative charge. So when the negatively charged electrons flow in a stream – that’s considered an electric current. Or when electrons build up on an unmoving surface – that’s considered static electricity. (It’s called “static” because it doesn’t move.)
One other important thing to know about protons and electrons is that they are attracted to each other. When an atom has equal numbers of electrons and protons, all of the positive charges and negative charges cancel each other out, leaving the overall charge neutral. If an atom has more electrons than protons, it is negatively charged, and, of course, if there are more protons than electrons, it is positively charged. Neither of those situations is very stable – atoms like to be neutral. So a negatively charged atom will try to find a positively charged atom and stick to it.
So if atoms like to have their protons and electrons balanced out, how do you get a buildup of electrons on a surface to make static electricity? When clothes are tumbling in a dryer, they are rubbing together like crazy in a warm, dry environment. This heat and friction makes the charges on the surface of the clothes separate. Take a look at this diagram, which shows the separation of charge on a sock. Some socks will get extra electrons, and other socks will lose their electrons. This is an unstable situation for the socks. The atoms want to regain their neutral charge.
So as the socks are tumbling in the dryer, a sock with a negative charge will find another sock with a positive charge and stick to it. Now when you pull the socks out of the dryer, they will remain stuck. It doesn’t have to be socks, of course. It could be sleeves of the same shirt, folds of a skirt, shirts to each other, underwear to pants. They will stick together. Voila. Static Cling.
So let’s go back to dryer sheets. Dryer sheets reduce this buildup of static electricity by releasing extra protons into the dryer during the dry cycle. These extra protons stick to the negatively charged clothes that are building up. And because these negatively charged clothes are now balanced with extra protons, they no longer stick to other pieces of clothing. So you get a nice, non-sticky load of warm, dry clothes at the end of the cycle.
This is a perfect illustration of how science can be found everywhere you look – even in something as mundane as doing the laundry. And I think we should all give a big hand to the science of static electricity – and those who figured out a way eliminate it.
I have. I tend to collect static electricity - my hair, my clothes, even my cats. So I absolutely have to have a dryer sheet in the dryer whenever I’m doing a load of laundry. If I don’t, I’m doomed to be a crackling, zapping, static-y mess. So in goes a dryer sheet, and out comes nice, static-free laundry. It’s like magic!
Well, actually, it isn't magic, it's science. Here’s how they work.
First, let’s talk about static electricity. Static electricity occurs when an electrical charge builds up on a surface. Electrical charge is made of electrons. (“Electrons” and “electricity” sound so similar because they are from the same root.) What are electrons? They are one of three major particles that make up atoms, along with protons and neutrons. The names of these things refer to the electrical charge that they carry: neutrons are neutral – not charged; protons have a positive charge; electrons have a negative charge. So when the negatively charged electrons flow in a stream – that’s considered an electric current. Or when electrons build up on an unmoving surface – that’s considered static electricity. (It’s called “static” because it doesn’t move.)
One other important thing to know about protons and electrons is that they are attracted to each other. When an atom has equal numbers of electrons and protons, all of the positive charges and negative charges cancel each other out, leaving the overall charge neutral. If an atom has more electrons than protons, it is negatively charged, and, of course, if there are more protons than electrons, it is positively charged. Neither of those situations is very stable – atoms like to be neutral. So a negatively charged atom will try to find a positively charged atom and stick to it.
So if atoms like to have their protons and electrons balanced out, how do you get a buildup of electrons on a surface to make static electricity? When clothes are tumbling in a dryer, they are rubbing together like crazy in a warm, dry environment. This heat and friction makes the charges on the surface of the clothes separate. Take a look at this diagram, which shows the separation of charge on a sock. Some socks will get extra electrons, and other socks will lose their electrons. This is an unstable situation for the socks. The atoms want to regain their neutral charge.
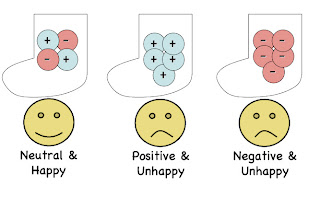
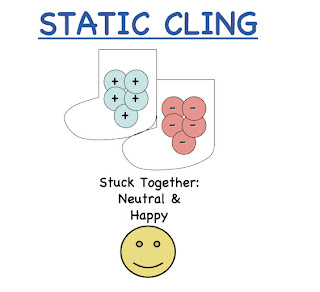
This is a perfect illustration of how science can be found everywhere you look – even in something as mundane as doing the laundry. And I think we should all give a big hand to the science of static electricity – and those who figured out a way eliminate it.
Thursday, January 17, 2008
The chemistry of fire
One of my favorite activities on a chilly winter night is to snuggle on the couch in front of a roaring fire in the fireplace. There’s something so cozy about it! Now that I think about it, fire is kind of a strange thing. It gives warmth and light – but it can also be highly destructive. It can sustain life – or it can destroy it. But what is it? When I have wood burning in my fireplace, what exactly is going on? What is fire?
Fire isn’t a thing. It’s a chemical reaction. Specifically, fire is a chemical reaction that releases heat and light energy as matter changes from one state to another.
Okay, sounds good. But what does it mean?
Let’s deal first with the first part of that definition – it’s a chemical reaction. Chemical reactions are wide and varied, and include everything from nails rusting to the bubbling of alka-seltzer tablets in a glass of water to the metabolism of sugars in your cells to produce energy. A chemical reaction simply means that 2 or more things (molecules, ions or atoms) interact and change to something else. So let’s look at a nail turning rusty. This is an example of an oxidation reaction. When iron is exposed to water and oxygen, the water and carbon dioxide in the air form a weak acid (called carbonic acid). Carbonic acid dissolves the iron, and also breaks more water down into hydrogen and oxygen. Free oxygen (from the water) and dissolved iron (from the nail) combine to form iron oxide – which is rust. The net result is that iron has changed into iron oxide - a completely different molecule than it was when you started.
So what is the chemical reaction that occurs in fire? For fire to occur, you must have 3 things - something to burn, oxygen and an ignition. So let’s look at the burning of wood. The ignition spark – which can come from any number of places (such as a match, a lightning bolt, or friction) - must bring the wood to about 300 degrees Fahrenheit. At this temperature, the cellulose in the wood begins to break apart. This breaking of the cellulose releases three things - volatile gases (namely oxygen, hydrogen and carbon), char (which is pure carbon) and ash (all the rest of the unburnable minerals from the wood). We see the volatile gases as smoke. For the wood to catch in flames, the temperature must now get to 500 degrees Fahrenheit. Now the molecules in the cellulose start to break apart into atoms. The atoms recombine with oxygen, forming water, carbon dioxide, and other stuff. The recombining of those atoms into new molecules produces a lot of energy, which takes the form of heat and light.
So you start with wood, heat and oxygen – and you wind up with water vapor, ash, and smoke. And all of the heat and light energy that is released in the transformation? That’s fire.
So the next time we have a fire in our fireplace, I hope that I can remember that this process of fire is really a great example of some fundamental chemistry. Maybe then I won’t take it for granted quite so much!
Fire isn’t a thing. It’s a chemical reaction. Specifically, fire is a chemical reaction that releases heat and light energy as matter changes from one state to another.
Okay, sounds good. But what does it mean?
Let’s deal first with the first part of that definition – it’s a chemical reaction. Chemical reactions are wide and varied, and include everything from nails rusting to the bubbling of alka-seltzer tablets in a glass of water to the metabolism of sugars in your cells to produce energy. A chemical reaction simply means that 2 or more things (molecules, ions or atoms) interact and change to something else. So let’s look at a nail turning rusty. This is an example of an oxidation reaction. When iron is exposed to water and oxygen, the water and carbon dioxide in the air form a weak acid (called carbonic acid). Carbonic acid dissolves the iron, and also breaks more water down into hydrogen and oxygen. Free oxygen (from the water) and dissolved iron (from the nail) combine to form iron oxide – which is rust. The net result is that iron has changed into iron oxide - a completely different molecule than it was when you started.
So what is the chemical reaction that occurs in fire? For fire to occur, you must have 3 things - something to burn, oxygen and an ignition. So let’s look at the burning of wood. The ignition spark – which can come from any number of places (such as a match, a lightning bolt, or friction) - must bring the wood to about 300 degrees Fahrenheit. At this temperature, the cellulose in the wood begins to break apart. This breaking of the cellulose releases three things - volatile gases (namely oxygen, hydrogen and carbon), char (which is pure carbon) and ash (all the rest of the unburnable minerals from the wood). We see the volatile gases as smoke. For the wood to catch in flames, the temperature must now get to 500 degrees Fahrenheit. Now the molecules in the cellulose start to break apart into atoms. The atoms recombine with oxygen, forming water, carbon dioxide, and other stuff. The recombining of those atoms into new molecules produces a lot of energy, which takes the form of heat and light.
So you start with wood, heat and oxygen – and you wind up with water vapor, ash, and smoke. And all of the heat and light energy that is released in the transformation? That’s fire.
So the next time we have a fire in our fireplace, I hope that I can remember that this process of fire is really a great example of some fundamental chemistry. Maybe then I won’t take it for granted quite so much!
Monday, January 14, 2008
Diseases you never want to get
I recently received a tetanus booster shot. Actually, it was called DTaP, and it was a combination vaccine against tetanus, diphtheria and pertussis. As I recovered from the booster shot (I had quite a sore arm for several days), I started wondering about these diseases. I wanted to know: what, biologically speaking, are they, anyways?
Let’s start with tetanus. Tetanus is caused by a soil-dwelling bacterium named Clostridium tetani. It can enter your body through a deep tissue wound that gets dirt in it (the classic example is stepping on a rusty nail). Other injuries involving dead skin, however, such as frostbite or burns, can also allow the bacterium a way into your system. There is also a form of neonatal tetanus, which can occur when a baby is delivered under unsanitary conditions. Once the bacterium is in the body, it produces a neurotoxin called tetanus toxin, which acts as a poison on your neurons. It stops the ability of the nerves controlling your skeletal muscles to turn themselves off; the risk is that these nerves will be firing signals at the smallest stimulus. This means that a small touch on your skin that would normally be ignored by your nervous system will result in a large, spastic muscle movement. The classic symptom of the disease is lockjaw, though other symptoms include difficulty swallowing, severe muscle spasms, and seizures. The spasms may continue up to 4 weeks, unless death occurs (in approximately 10-20% of the cases).
Sounds dreadful, doesn’t it? So here’s some good news: vaccination against tetanus is very effective. Even if you are behind on your tetanus boosters, there is even a post-exposure tetanus prophylaxis that you will receive if needed. Because of this, tetanus is very rare in the US. There have been fewer than 40 cases per year in the US since 1999. Phew!
How about diphtheria? Diphtheria is caused by Corynebacterium diphtheriae, There are 2 types of diphtheria – one in the upper respiratory tract, and one on the skin. The cutaneous (meaning skin) form of diphtheria is usually a secondary infection of a pre-existing skin wound. It is considered a mild form of the disease. Respiratory diptheria, however, can be very severe. It is spread very easily through respiratory droplets (such as those made by a cough or a sneeze) of an infected individual. The disease manifests as a sore throat, low-grade fever, swollen glands, and weakness. The most prominent symptom, however, is the accumulation of a thick, gray membrane over the throat and tonsils. This membrane can make swallowing and breathing difficult. Once established in the respiratory system, it can also spread to other organs, such as the heart and kidney. One in ten infected individuals will ultimately die from such an infection.
Corynebacterium diphtheriae is scientifically very interesting. It colonizes local tissues of the throat, then produces a toxin called diphtheria toxin, which interferes with a cell’s ability to make protein. This interference is lethal; any such cell affected by the toxin will die. There are actually 3 different substrains of this bacteria, each of which is capable of producing different amounts of toxin. The gravis strain can produce the most toxin, thus it causes the most severe form of the disease. However, toxin production is controlled not only by the genetics of the bacterium, but also the environment of the throat. In particular, inorganic iron prevents the synthesis of the toxin. So if you don’t have much iron in your bloodstream, the bacterium can produce much more toxin.
I know, this sounds horrible, too. But here’s the good news: diphtheria is also very rare in the US, again due to good vaccination protocols. There are fewer than 10 cases reported across the country every year. If someone does become infected, treatment options are pretty good. Immediate hospitalization and treatment allows most patients to recover from the disease.
And for the final disease of the day – pertussis. You may know pertussis better as whooping cough. This is a highly contagious disease of the upper respiratory tract. It is caused by the bacterium Bordetella pertussis, which lives in the mouth, nose and throat of mammals; there is no other known environment in which is bacterium is found. It is pathogenic only in humans. B. pertussis causes this disease because it, too, produces a toxin. Petussis toxin alters a cell’s ability to regulate internal levels of a molecule called cAMP. Elevated cAMP levels in a cell results in it losing fluid and ions. Pertussis toxin also has a very specific effect on cells of your immune system called phagocytes. Phagocytes normally engulf and destroy foreign matter in your body, such as bacteria. However, phagocytes affected by pertussis toxin can no work in this way, and a primary line of defense against the B. pertussis bacterium is lost.
The outcome of infection progresses in several stages. The first stage resembles a simple cold – runny nose, sneezing, low-grade fever and mild cough. The second stage is when the disease is at its worst. The cough becomes very severe. Infected individuals will have uncontrollable fits of coughing, following by a characteristic high-pitched crowing, or “whoop,” as the person breathes in. (This is why it is commonly called whooping cough.) The coughing fits can be so violent that they can cause the person to turn blue from lack of oxygen, or they may vomit once it is over. Between coughing episodes, the person will appear normal; however, they can count on around 20 such fits a day. The third phase of pertussis is the recovery phase, during which symptoms gradually disappear. There are many possible complications from pertussis, including pneumonia, ear infections, dehydration, seizures, encephalopathy (abnormal brain function due to oxygen deprivation) and death. The disease is most severe in young children; older children and adults usually have an easier time of it.
I was very surprised to learn about the frequency of pertussis in the US. Cases reached an all-time low in 1976 (1,010 cases reported); but that number has been slowly rising. According to the CDC, 5000 to 7000 cases of the disease are reported every year. One of the reasons for this is that immunity from infection or vaccination is not life-long; boosters are required to maintain immunity. But because it is so rapidly transmitted, anyone behind on their boosters can get a mild form of the disease, which is then transmitted. It is believed that more of these mild cases are now being recognized, whereas they would have previously been treated as a regular respiratory infection. There is some good news, though – treatment for established cases of pertussis is very good, and the number of reported deaths is very small.
After learning about all of this, I’m really glad that I was recently vaccinated against all of these things! None of them are anything I’d ever want to contract.
Let’s start with tetanus. Tetanus is caused by a soil-dwelling bacterium named Clostridium tetani. It can enter your body through a deep tissue wound that gets dirt in it (the classic example is stepping on a rusty nail). Other injuries involving dead skin, however, such as frostbite or burns, can also allow the bacterium a way into your system. There is also a form of neonatal tetanus, which can occur when a baby is delivered under unsanitary conditions. Once the bacterium is in the body, it produces a neurotoxin called tetanus toxin, which acts as a poison on your neurons. It stops the ability of the nerves controlling your skeletal muscles to turn themselves off; the risk is that these nerves will be firing signals at the smallest stimulus. This means that a small touch on your skin that would normally be ignored by your nervous system will result in a large, spastic muscle movement. The classic symptom of the disease is lockjaw, though other symptoms include difficulty swallowing, severe muscle spasms, and seizures. The spasms may continue up to 4 weeks, unless death occurs (in approximately 10-20% of the cases).
Sounds dreadful, doesn’t it? So here’s some good news: vaccination against tetanus is very effective. Even if you are behind on your tetanus boosters, there is even a post-exposure tetanus prophylaxis that you will receive if needed. Because of this, tetanus is very rare in the US. There have been fewer than 40 cases per year in the US since 1999. Phew!
How about diphtheria? Diphtheria is caused by Corynebacterium diphtheriae, There are 2 types of diphtheria – one in the upper respiratory tract, and one on the skin. The cutaneous (meaning skin) form of diphtheria is usually a secondary infection of a pre-existing skin wound. It is considered a mild form of the disease. Respiratory diptheria, however, can be very severe. It is spread very easily through respiratory droplets (such as those made by a cough or a sneeze) of an infected individual. The disease manifests as a sore throat, low-grade fever, swollen glands, and weakness. The most prominent symptom, however, is the accumulation of a thick, gray membrane over the throat and tonsils. This membrane can make swallowing and breathing difficult. Once established in the respiratory system, it can also spread to other organs, such as the heart and kidney. One in ten infected individuals will ultimately die from such an infection.
Corynebacterium diphtheriae is scientifically very interesting. It colonizes local tissues of the throat, then produces a toxin called diphtheria toxin, which interferes with a cell’s ability to make protein. This interference is lethal; any such cell affected by the toxin will die. There are actually 3 different substrains of this bacteria, each of which is capable of producing different amounts of toxin. The gravis strain can produce the most toxin, thus it causes the most severe form of the disease. However, toxin production is controlled not only by the genetics of the bacterium, but also the environment of the throat. In particular, inorganic iron prevents the synthesis of the toxin. So if you don’t have much iron in your bloodstream, the bacterium can produce much more toxin.
I know, this sounds horrible, too. But here’s the good news: diphtheria is also very rare in the US, again due to good vaccination protocols. There are fewer than 10 cases reported across the country every year. If someone does become infected, treatment options are pretty good. Immediate hospitalization and treatment allows most patients to recover from the disease.
And for the final disease of the day – pertussis. You may know pertussis better as whooping cough. This is a highly contagious disease of the upper respiratory tract. It is caused by the bacterium Bordetella pertussis, which lives in the mouth, nose and throat of mammals; there is no other known environment in which is bacterium is found. It is pathogenic only in humans. B. pertussis causes this disease because it, too, produces a toxin. Petussis toxin alters a cell’s ability to regulate internal levels of a molecule called cAMP. Elevated cAMP levels in a cell results in it losing fluid and ions. Pertussis toxin also has a very specific effect on cells of your immune system called phagocytes. Phagocytes normally engulf and destroy foreign matter in your body, such as bacteria. However, phagocytes affected by pertussis toxin can no work in this way, and a primary line of defense against the B. pertussis bacterium is lost.
The outcome of infection progresses in several stages. The first stage resembles a simple cold – runny nose, sneezing, low-grade fever and mild cough. The second stage is when the disease is at its worst. The cough becomes very severe. Infected individuals will have uncontrollable fits of coughing, following by a characteristic high-pitched crowing, or “whoop,” as the person breathes in. (This is why it is commonly called whooping cough.) The coughing fits can be so violent that they can cause the person to turn blue from lack of oxygen, or they may vomit once it is over. Between coughing episodes, the person will appear normal; however, they can count on around 20 such fits a day. The third phase of pertussis is the recovery phase, during which symptoms gradually disappear. There are many possible complications from pertussis, including pneumonia, ear infections, dehydration, seizures, encephalopathy (abnormal brain function due to oxygen deprivation) and death. The disease is most severe in young children; older children and adults usually have an easier time of it.
I was very surprised to learn about the frequency of pertussis in the US. Cases reached an all-time low in 1976 (1,010 cases reported); but that number has been slowly rising. According to the CDC, 5000 to 7000 cases of the disease are reported every year. One of the reasons for this is that immunity from infection or vaccination is not life-long; boosters are required to maintain immunity. But because it is so rapidly transmitted, anyone behind on their boosters can get a mild form of the disease, which is then transmitted. It is believed that more of these mild cases are now being recognized, whereas they would have previously been treated as a regular respiratory infection. There is some good news, though – treatment for established cases of pertussis is very good, and the number of reported deaths is very small.
After learning about all of this, I’m really glad that I was recently vaccinated against all of these things! None of them are anything I’d ever want to contract.
Friday, January 11, 2008
Saturn’s flying saucers
Did you know that there are flying saucers around Saturn?
Okay, I confess, they’re not really flying saucers. They’re moons. But they sure look like flying saucers. At least, they look like the flying saucers from the space alien movies from the 1960s. You know, the ones that look like this:
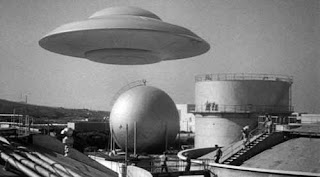
The 2 moons in question are called Pan and Atlas. They’re really small; Atlas is only about 25 miles by 15 miles in size. That’s tiny compared to the size of Saturn’s biggest moon, Titan, which has an equatorial radius of 1600 miles. (Incidentally, that makes Titan larger than the planet Mercury, and much bigger than the ex-planet Pluto.)
These small planets have confused scientists ever since they were discovered by the Voyager 1 and 2 spacecraft in the early 1980s. Recently, the Cassini spacecraft has provided highly detailed images of the moons, showing us how oddly-shaped they really are. A research team from the Space Science Institute in Boulder, Colorado, believes it may have the answer for why these moons have such an odd shape.
Saturn’s rings lie in a flat disc that corresponds to the planet’s equator; the moons are actually embedded within those rings. Atlas lies within the A-ring, the outer of Saturn’s 2 brightest rings. Pan is within the Encke Gap of the A-ring. (The diagram here shows the naming convention of the A- and B-rings, as well as the Encke Gap.)
Scientists had previously argued that the moons were made up solely of fragments left over from collisions of larger moons. However, scientists from a team led by Carolyn Porco have concluded that the moons are made up primarily of material just like the rings themselves – light, porous and icy. In fact, as much as two-thirds of the moons might consist of this matter, which is quite unlike the dense, massive material that would be the leftovers of other planetary bodies.
Porco’s research group believes that the cores of Pan and Atlas likely came from these planetary leftovers. But over the millennia, the moons may have drawn dust and ice slowly to themselves, building themselves up. Two important features of the moons are consistent with this model: the ridges around the equators of the moons are in the same plane as the rings of Saturn, and they are as thick as the vertical distance that the moons cover in the rings. So it makes sense that they could likely have picked up material as they orbited Saturn to make themselves larger. This accumulation would likely have occurred in several stages, the last of which would have resulted in the strange ridges that give them their flying saucer shape. That’s because the moons act as “accretion discs;” as matter falls towards a gravitational pull, it gradually builds up into discs. In fact, this is quite possibly comparable to how Saturn’s rings themselves were made.
However, it is also believed that Pan and Atlas have reached their final shape, and will not grow any larger. That’s because their orbits are thought to prevent any additional material from being able to stick stably to their surfaces.
But don’t worry. Despite appearances, Martians are not making their way towards Saturn in their flying saucers. I guess these are just another one of the bizarre things in our universe!
The following image was used in this entry:
Flying saucer: http://www.altfg.com/blog/biography-obit/bernard-gordon/
Okay, I confess, they’re not really flying saucers. They’re moons. But they sure look like flying saucers. At least, they look like the flying saucers from the space alien movies from the 1960s. You know, the ones that look like this:
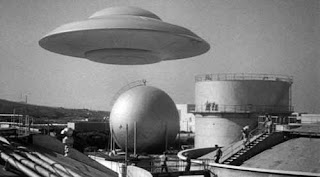
The 2 moons in question are called Pan and Atlas. They’re really small; Atlas is only about 25 miles by 15 miles in size. That’s tiny compared to the size of Saturn’s biggest moon, Titan, which has an equatorial radius of 1600 miles. (Incidentally, that makes Titan larger than the planet Mercury, and much bigger than the ex-planet Pluto.)
These small planets have confused scientists ever since they were discovered by the Voyager 1 and 2 spacecraft in the early 1980s. Recently, the Cassini spacecraft has provided highly detailed images of the moons, showing us how oddly-shaped they really are. A research team from the Space Science Institute in Boulder, Colorado, believes it may have the answer for why these moons have such an odd shape.
Saturn’s rings lie in a flat disc that corresponds to the planet’s equator; the moons are actually embedded within those rings. Atlas lies within the A-ring, the outer of Saturn’s 2 brightest rings. Pan is within the Encke Gap of the A-ring. (The diagram here shows the naming convention of the A- and B-rings, as well as the Encke Gap.)
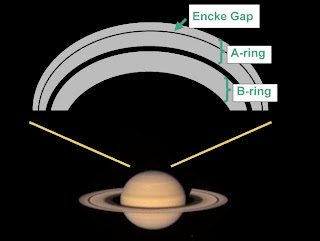
Porco’s research group believes that the cores of Pan and Atlas likely came from these planetary leftovers. But over the millennia, the moons may have drawn dust and ice slowly to themselves, building themselves up. Two important features of the moons are consistent with this model: the ridges around the equators of the moons are in the same plane as the rings of Saturn, and they are as thick as the vertical distance that the moons cover in the rings. So it makes sense that they could likely have picked up material as they orbited Saturn to make themselves larger. This accumulation would likely have occurred in several stages, the last of which would have resulted in the strange ridges that give them their flying saucer shape. That’s because the moons act as “accretion discs;” as matter falls towards a gravitational pull, it gradually builds up into discs. In fact, this is quite possibly comparable to how Saturn’s rings themselves were made.
However, it is also believed that Pan and Atlas have reached their final shape, and will not grow any larger. That’s because their orbits are thought to prevent any additional material from being able to stick stably to their surfaces.
But don’t worry. Despite appearances, Martians are not making their way towards Saturn in their flying saucers. I guess these are just another one of the bizarre things in our universe!
The following image was used in this entry:
Flying saucer: http://www.altfg.com/blog/biography-obit/bernard-gordon/
Monday, January 7, 2008
Something really weird about whales
Did you know that whales have earwax?
I discovered this fact while investigating human earwax (for my entry “Funny things about humans”). And as I thought about it a bit, I came up with a few questions about whales. What are some of the main features of a whale’s body? What kinds of features distinguish different whale species? What’s the smallest whale in the world? And do all whales have earwax?
There are 2 kinds of whales – baleen whales and toothed whales. Baleen whales are also known as whalebone whales, great whales, or Mysticeti. They are one of 2 suborders that make up Cetacea (that is, all whales, dolphins and porpoises). The main feature of these whales is their lack of teeth. Instead of teeth, they have baleen plates that filter food from water like a sieve. Baleen plates are composed mostly of a protein called keratin, and they are arranged in 2 parallel rows that grow out of the upper jaw. It looks like combs of thick hair. A baleen plate can be anywhere from 2 to 12 feet long, and weigh up to 90 pounds, and will grow continually throughout the lifespan of the whale, with the end continually wearing away. In contrast to baleen whales, toothed whales (also known as Odontoceti) have teeth
(though you probably had guessed that already). These teeth are mostly used for eating, though they can also be used for aggression. There is a wide variety in teeth among toothed whales. Some dolphins have over 100 teeth in the jaws, while the narwhal whale has a single tooth (which looks like a tusk protruding from the left side of the mouth).
Baleen whales and toothed whales also have different kinds of blowholes. A whale’s blowhole is analogous to our nostrils, the hole in the head through which the animal breathes. In contrast to mammals, though, a whale’s blowhole does not actually connect to the esophagus (the tube that food goes through from the mouth to the stomach). It only connects to the trachea (the windpipe). Because of this, a whale cannot breathe through its mouth. It must breathe through its blowhole on the top of its head. Baleen whales actually have 2 blowholes positioned in a V-shape, while toothed whales have a single blowhole.
Baleen whales tend to be larger than toothed whales; in fact, the largest mammal in the world is a baleen – the blue whale.
Other baleen whales include fin whales, minke whales, humpback whales, and gray whales. There are small baleen whales, too – the smallest is the pygmy right whale, which is around 20 feet long. The largest toothed whale is the sperm whale. At an average of 60 feet long, sperm whales are actually the largest toothed animals in the world. The sperm whale is unusual, though, as toothed whales tend to be small. Examples of toothed whales include dolphins, porpoises, narwhal whales, beaked whales, killer whales, pilot whales and beluga whales. The smallest whale in the world is the toothed dwarf sperm whale, otherwise known as the Owen’s pygmy sperm whale. These guys average around 8 feet long, weighing in at around 300 pounds. That makes them even smaller than dolphins.
As far as earwax goes, it has only been found in baleen whales. I have found references to earwax in both fin whales and humpback whales. Whale earwax serves a different purpose than our earwax. Its primary job is to prevent water from entering the ear canal, and it is not shed. In fact, baleen whales accumulate layers of wax as they get older; the more layers of earwax, the older the whale. Fin whales add 2 layers of wax a year, while humpback whales add 4. Scientists can use these layers to approximate the age of the whale. Actually, that’s the only way of aging a baleen whale. Toothed whales can be aged by looking at their teeth, because teeth grow in layers. You can count the layers of a whale’s tooth and see how it was. But baleen doesn’t grow that way. So the only way of knowing how old a baleen whale was is by counting the layers of its waxy ear plug.
I wonder if toothed whales also have earwax? They all have ears, which are just holes in the side of their heads immediately behind their eyes. And presumably they all need a way to keep water from flooding their ear canals. Maybe they have some other way of keeping water out of their ears. Or maybe we just haven’t looked hard enough yet?
The images I used were taken from the following sites:
Narwhal whale: http://concise.britannica.com/ebc/art-6694/Narwhal
Blue whale: http://en.wikibooks.org/wiki/Adventist_Youth_Honors_Answer_Book/
Nature/Marine_Mammals
I discovered this fact while investigating human earwax (for my entry “Funny things about humans”). And as I thought about it a bit, I came up with a few questions about whales. What are some of the main features of a whale’s body? What kinds of features distinguish different whale species? What’s the smallest whale in the world? And do all whales have earwax?
There are 2 kinds of whales – baleen whales and toothed whales. Baleen whales are also known as whalebone whales, great whales, or Mysticeti. They are one of 2 suborders that make up Cetacea (that is, all whales, dolphins and porpoises). The main feature of these whales is their lack of teeth. Instead of teeth, they have baleen plates that filter food from water like a sieve. Baleen plates are composed mostly of a protein called keratin, and they are arranged in 2 parallel rows that grow out of the upper jaw. It looks like combs of thick hair. A baleen plate can be anywhere from 2 to 12 feet long, and weigh up to 90 pounds, and will grow continually throughout the lifespan of the whale, with the end continually wearing away. In contrast to baleen whales, toothed whales (also known as Odontoceti) have teeth
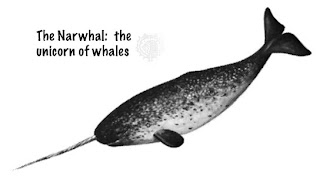
Baleen whales and toothed whales also have different kinds of blowholes. A whale’s blowhole is analogous to our nostrils, the hole in the head through which the animal breathes. In contrast to mammals, though, a whale’s blowhole does not actually connect to the esophagus (the tube that food goes through from the mouth to the stomach). It only connects to the trachea (the windpipe). Because of this, a whale cannot breathe through its mouth. It must breathe through its blowhole on the top of its head. Baleen whales actually have 2 blowholes positioned in a V-shape, while toothed whales have a single blowhole.
Baleen whales tend to be larger than toothed whales; in fact, the largest mammal in the world is a baleen – the blue whale.
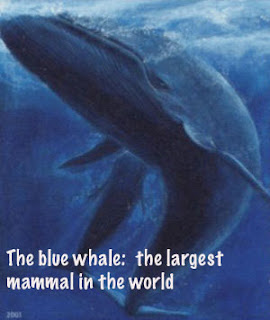
As far as earwax goes, it has only been found in baleen whales. I have found references to earwax in both fin whales and humpback whales. Whale earwax serves a different purpose than our earwax. Its primary job is to prevent water from entering the ear canal, and it is not shed. In fact, baleen whales accumulate layers of wax as they get older; the more layers of earwax, the older the whale. Fin whales add 2 layers of wax a year, while humpback whales add 4. Scientists can use these layers to approximate the age of the whale. Actually, that’s the only way of aging a baleen whale. Toothed whales can be aged by looking at their teeth, because teeth grow in layers. You can count the layers of a whale’s tooth and see how it was. But baleen doesn’t grow that way. So the only way of knowing how old a baleen whale was is by counting the layers of its waxy ear plug.
I wonder if toothed whales also have earwax? They all have ears, which are just holes in the side of their heads immediately behind their eyes. And presumably they all need a way to keep water from flooding their ear canals. Maybe they have some other way of keeping water out of their ears. Or maybe we just haven’t looked hard enough yet?
The images I used were taken from the following sites:
Narwhal whale: http://concise.britannica.com/ebc/art-6694/Narwhal
Blue whale: http://en.wikibooks.org/wiki/Adventist_Youth_Honors_Answer_Book/
Nature/Marine_Mammals
Friday, January 4, 2008
The Permian Extinction
In a recent entry (“A super-sized bug”), I mentioned that ancient sea scorpions disappeared from the fossil record around 250 million years ago, during a time known as the Permian extinction. What was the Permian extinction? In short, it was one of the most cataclysmic mass extinction events ever to happen to life on earth. There have actually been 5 massive extinctions over the earth’s history. They are the Ordovician extinction (440 million years ago), the Devonian extinction (370 million years ago), the Permian extinction (250 million years ago), the Triassic extinction (210 million years ago) and the Cretaceous extinction (65 million years ago). The Permian was by far the biggest. In total, 70% of all land-based species became extinct; 95% of all marine life was killed. It has been referred to as “the mother of all mass extinctions.”
The Permian period lasted from approximately 286 to 248 million years ago, and was the last period of the Paleozoic era (which lasted from 540 to 248 million years ago). During the Permian period, all of the earth’s continents were in 1 giant land mass. It was called Pangea; shaped like the letter “C,” it spanned the equator. The mouth of the C was a sea called Tethys, while the rest of the world was covered by a giant ocean called Panthalassa.
This configuration of land and ocean led to an expansion and diversification of both terrestrial and marine species. Therapsids were the dominant land-based tetrapod (which means 4-legged creature), and have also been called “mammal-like reptiles.” There were multiple kinds of therapsids, including dinocephalians (a dog-like crocodile), gorgonopsians (a large reptilian saber-toothed tiger), therocephalia (smaller than the gorgonopsians), and dicynodonts (the only herbivores in the group). There were also several primitive turtle-like and reptilian-like creatures, including pareisaurs (armoured herbivores reaching a whopping 9 feet long), procolophonids (chunky lizards with big skulls), and diapsids (which still exist in the form of reptiles and birds). Dominant insects were mostly cockroaches (upwards of 90% of insects were some kind of roach), though dragonflies were also abundant. Terrestrial flora changed dramatically during this time. Pangea had large areas of relatively dry land, as opposed to the damp, jungle-like climate that had existed previously. Swamp-loving trees were replaced with conifers (pine trees), and gingkos, cycads and ferns also become prominent. The marine environment was full of exotic life. Great reefs harbored giant sea scorpions, armoured fish, mollusks, sponges, trilobites, brachiopods (bivalves that look like clams), nautiloids (to which the modern nautilus belongs), and ammonoids (related to octopus and squid).
The organisms of Pangea during the Permian period were vast and varied. But after the Permian extinction, very few of these creatures had survived. What happened to destroy so much of this varied and exotic life? At the moment, there are several theories to explain the massive die-off.
One theory is that massive volcanic eruptions coated the landscape of the present-day Siberian Traps with basalt lava, causing environmental stress, an elevation of the earth’s temperature, and mass extinction. An expansion of this theory says that the increased temperature resulting from the basalt lava would not be enough to destroy so much life. But it could result in the release of methane gas from below the ocean floor, which would further increase global temperatures. Yet another related theory is that the basalt lava decreased the ability of the oceans to dissolve oxygen. Because of this, deep-ocean bacteria thrived (they don’t use oxygen), and they produced massive amounts of hydrogen sulfide. The hydrogen sulfide escaped into the atmosphere, causing acid rain and destroying the ozone layer. In fact, recent evidence from David Bottjer, professor of earth and biological sciences from the University of Southern California, supports this model of an uprising of hydrogen sulfide from the deep ocean. In a paper published in the November issue of the journal Geology, Dr. Bottjer reported evidence that deep-sea organisms were the first to die off, followed only later by creatures dwelling in shallow water. Many people also believe that this model could explain the later Cretaceous extinction event that wiped out the dinosaurs. (That was the second largest extinction event in the world.)
Whatever the cause, the effects on life on earth were nothing short of disastrous. While 70% of land species and 95% of marine species died out during this extinction event, on an individual level, perhaps as many as 99% of all individual organisms died as a result of whatever happened. This clearing of the landscape provided room for the emergence of other well-known species, including the dinosaurs and modern mammals. So in retrospect, it wasn’t a total loss. But it certainly was a tough time for everything involved.
The Permian period lasted from approximately 286 to 248 million years ago, and was the last period of the Paleozoic era (which lasted from 540 to 248 million years ago). During the Permian period, all of the earth’s continents were in 1 giant land mass. It was called Pangea; shaped like the letter “C,” it spanned the equator. The mouth of the C was a sea called Tethys, while the rest of the world was covered by a giant ocean called Panthalassa.
This configuration of land and ocean led to an expansion and diversification of both terrestrial and marine species. Therapsids were the dominant land-based tetrapod (which means 4-legged creature), and have also been called “mammal-like reptiles.” There were multiple kinds of therapsids, including dinocephalians (a dog-like crocodile), gorgonopsians (a large reptilian saber-toothed tiger), therocephalia (smaller than the gorgonopsians), and dicynodonts (the only herbivores in the group). There were also several primitive turtle-like and reptilian-like creatures, including pareisaurs (armoured herbivores reaching a whopping 9 feet long), procolophonids (chunky lizards with big skulls), and diapsids (which still exist in the form of reptiles and birds). Dominant insects were mostly cockroaches (upwards of 90% of insects were some kind of roach), though dragonflies were also abundant. Terrestrial flora changed dramatically during this time. Pangea had large areas of relatively dry land, as opposed to the damp, jungle-like climate that had existed previously. Swamp-loving trees were replaced with conifers (pine trees), and gingkos, cycads and ferns also become prominent. The marine environment was full of exotic life. Great reefs harbored giant sea scorpions, armoured fish, mollusks, sponges, trilobites, brachiopods (bivalves that look like clams), nautiloids (to which the modern nautilus belongs), and ammonoids (related to octopus and squid).
The organisms of Pangea during the Permian period were vast and varied. But after the Permian extinction, very few of these creatures had survived. What happened to destroy so much of this varied and exotic life? At the moment, there are several theories to explain the massive die-off.
One theory is that massive volcanic eruptions coated the landscape of the present-day Siberian Traps with basalt lava, causing environmental stress, an elevation of the earth’s temperature, and mass extinction. An expansion of this theory says that the increased temperature resulting from the basalt lava would not be enough to destroy so much life. But it could result in the release of methane gas from below the ocean floor, which would further increase global temperatures. Yet another related theory is that the basalt lava decreased the ability of the oceans to dissolve oxygen. Because of this, deep-ocean bacteria thrived (they don’t use oxygen), and they produced massive amounts of hydrogen sulfide. The hydrogen sulfide escaped into the atmosphere, causing acid rain and destroying the ozone layer. In fact, recent evidence from David Bottjer, professor of earth and biological sciences from the University of Southern California, supports this model of an uprising of hydrogen sulfide from the deep ocean. In a paper published in the November issue of the journal Geology, Dr. Bottjer reported evidence that deep-sea organisms were the first to die off, followed only later by creatures dwelling in shallow water. Many people also believe that this model could explain the later Cretaceous extinction event that wiped out the dinosaurs. (That was the second largest extinction event in the world.)
Whatever the cause, the effects on life on earth were nothing short of disastrous. While 70% of land species and 95% of marine species died out during this extinction event, on an individual level, perhaps as many as 99% of all individual organisms died as a result of whatever happened. This clearing of the landscape provided room for the emergence of other well-known species, including the dinosaurs and modern mammals. So in retrospect, it wasn’t a total loss. But it certainly was a tough time for everything involved.
Subscribe to:
Posts (Atom)